Effects of pimobendan on myocardial perfusion and pulmonary transit time in dogs with myxomatous mitral valve disease: a pilot study
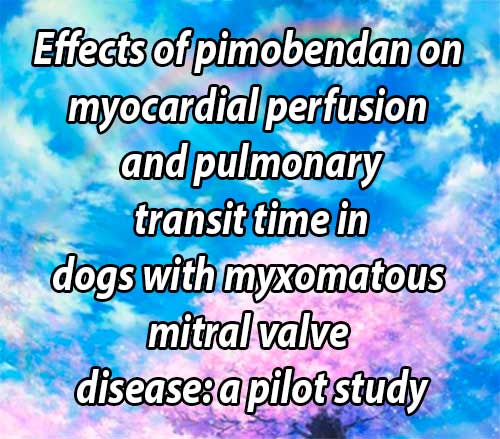
Author information
Apple S.M., Menciotti G., Braz-Ruivo L., Crosara S., Häggström J., Borgarelli M. Effects of pimobendan on myocardial perfusion and pulmonary transit time in dogs with myxomatous mitral valve disease: a pilot study // Aust Vet J. 2016 Sep;94(9):324-8.
Abstract
OBJECTIVES: To describe pulmonary transit time (nPTT) and myocardial perfusion (nMP) normalised to heart rate in dogs with stable ACVIM stage C myxomatous mitral valve disease (MMVD) and to assess short-term effects of pimobendan on these variables. We hypothesised that nPTT and nMP would increase in dogs with myxomatous mitral valve disease compared with normal dogs. Additionally, we hypothesised that treatment with pimobendan would decrease nMP and nPTT in dogs with MMVD.
DESIGN: Prospective, single-blind study involving 6 normal dogs and 12 dogs with MMVD.
METHODS: Dogs with MMVD were treated with enalapril and furosemide for at least 1 month prior to examination. All dogs underwent standard and contrast echocardiographic examinations at the beginning of the study (T0). At this time, myxomatous mitral valve disease dogs were randomly assigned to receive either pimobendan (0.4-0.6 mg/kg) or not. All dogs with MMVD were re-evaluated by standard and contrast echocardiography after 1 week (T1) and nPTT and nMP were measured.
RESULTS: nPTT was significantly increased in dogs with myxomatous mitral valve disease (P = 0.0063), compared with normal dogs. It was significantly decreased at T1 in dogs receiving pimobendan (P = 0.0250). The nMP was not significantly different in dogs with myxomatous mitral valve disease, compared with healthy dogs (P = 0.2552), and it was not significantly different at T1 in the treatment group (P = 0.8798).
CONCLUSIONS: Contrast echocardiography was a valid, complementary tool for echocardiographic analysis of dogs with MMVD. Pimobendan decreased nPTT in dogs affected by MMVD. Myocardial perfusion was not different in dogs with severe myxomatous mitral valve disease.
Echocardiographic contrast agents are characterised by stable particles smaller than red blood cells and capable of crossing the capillaries of the lungs and entering the left heart when injected into a peripheral vein, thus allowing for the measurement of pulmonary transit time (PTT).1 The particles expand and implode when their linear flow is disrupted via high acoustic pressure ultrasound, a process known as cavitation.2 This feature, together with the capability of these particles to enter the small capillaries of the myocardium, also allows for evaluation of myocardial perfusion (MP).3
A limited number of studies have shown that these contrast agents are safe and allow for accurate echocardiographic measurements in normal dogs and cats.4,5 Echocardiographic contrast also increases the opacity of the cardiac chambers and strengthens the Doppler signal, allowing clinicians to better identify endocardial borders and fluid dynamics via non-invasive visualisation.3,6 Troponin-I (cTnI) has been shown to be a sensitive and specific measure of myocardial damage in veterinary patients and is commonly used to measure cardiac ischaemic damage in humans.7,8 Although myocardial damage by cavitation following rupture of microbubbles is a common concern, contrast echocardiography does not appear to increase the levels of cTnI in normal dogs.4 Although the utility of cardiac contrast agents appear promising, their use has been limited to research applications in canine and feline patients. Myxomatous mitral valve disease (MMVD) associated with congestive heart failure (CHF) has been shown to lead to myocardial fibrosis and arterial changes within the myocardium based on postmortem analysis.
Therefore, measurement of MP in the clinical setting might allow clinicians to better assess the progression of MMVD in dogs.9,10 PTT has been reported to represent a generic index of cardiovascular function and, as assessed by radionuclide angiography, has been shown to be increased in dogs with MMVD.11 Pimobendan is a dual-action inodilator that is commonly prescribed to dogs with MMVD and CHF.12 There are two important effects of pimobendan that dogs with myxomatous mitral valve disease may benefit from. Firstly, inotropes increase the contractility of the heart by increasing calciumchannel sensitivity; secondly, pimobendan decreases cardiac preload and afterload by phosphodiesterase III inhibition, resulting in vasodilation.13 Pimobendan has also been reported to increase MP and survival in animals suffering from heart disease and CHF,14–16 and has been shown to decrease left atrial pressures, when given at a low dose to dogs with experimentally induced MV chord rupture
We have previously reported the feasibility and safety of using echocardiographic contrast agents in healthy dogs.4 In order to expand upon the utility of echocardiographic contrast agents, we aimed to validate their efficacy in visualising canine hearts with myxomatous mitral valve disease by comparing normalised PTT (nPTT) to normalised MP (nMP) in normal dogs and dogs affected with MMVD. We hypothesised that nPTT and nMP would be increased in dogs with stable stage C ACVIM MMVD compared with normal dogs.18 Additionally, we hypothesised that treatment with pimobendan would decrease nMP and nPTT in dogs with myxomatous mitral valve disease.
Materials and methods
We included 18 client-owned dogs: 6 healthy dogs and 12 dogs with ACVIM stage myxomatous mitral valve disease. There were no restrictions on breed or sex of the dogs. Diagnosis of stage C myxomatous mitral valve disease was based on the presence of a left apical systolic murmur on physical examination, echocardiographic evidence of thickening of the MV leaflets and evidence of MV regurgitation on colour Doppler. Dogs in the latter group also had a normalised left ventricular end-diastolic dimension > 1.7, a 2D left atrium to aortic root ratio (LA/Ao) > 1.7 and presented with current or past signs of CHF (e.g. exercise intolerance, dyspnoea and coughing) and documented current or past evidence of pulmonary oedema on thoracic radiographs.19 Dogs had to weigh ≤ 20 kg to be included in this study. Animals were excluded from the study if they showed clinical and/or echocardiographic evidence of cardiac disease (congenital or acquired) other than myxomatous mitral valve disease. Dogs with evidence of other significant systemic or organ-related disease, such as liver, kidney or gastrointestinal diseases, assessed by means of clinical evaluation, serum biochemistry or both were not included in the study.
Patients were excluded if they had a systolic blood pressure > 180 mmHg assessed by Doppler-cuff method or if their body weight was > 20 kg. All dogs with MMVD were treated with furosemide (1–4 mg/kg twice daily) and enalapril (0.5 mg/kg twice daily) for at least 4 weeks prior to study, without changing the dose. These drugs were continued during the study period. On the day of enrolment (T0), 6 of the 12 dogs with myxomatous mitral valve disease were randomly assigned to receive pimobendan at a dose of 0.4–0.6 mg/kg daily, in addition to their ongoing therapy. At 1 week after (T1), pimobendan was prescribed to all MMVD dogs. One dog died suddenly prior to followup and was excluded from analysis of the effect of the treatment, but was included in the initial evaluation of myxomatous mitral valve disease patients with contrast echocardiography. This dog was in the non-pimobendan treatment group. This study was conducted in a single-blinded manner.
To keep the investigator blinded to the study, a dispenser was used to supply the medication to the owners at each study site, based on a predetermined randomisation code. Each owner was instructed to avoid any reference to the treatment at the time of the recheck. The study was approved by the Kansas State University’s IACUC committee (No. 2818). Standard and contrast echocardiographic images were acquired at T0 and T1 for all dogs by two board-certified cardiologists using two cardiovascular ultrasonographic systems (Vivid 7 Dimension cardiovascular ultrasound system BT 2006, GE Healthcare, Milwaukee, WI, USA; iE33, Philips Ultrasound, Andover, MA, USA) equipped with matrix array transducers (M4S matrix array transducer, GE Healthcare; S4 matrix array transducer, Philips Ultrasound). The LA/Ao was assessed as previously reported.20 Left ventricular ejection fraction was measured by the area–length method from the left parasternal apical 4-chamber view.21 Contrast examinations were performed using the contrast imaging dedicated application embedded in the ultrasound machines. This is a low-power, real-time technique based on pulse inversion combined with Doppler ultrasonography at a frame rate of 20 Hz. With this application and a contrast agent, the optimal agent-to-tissue ratio can be achieved with a mechanical index (MI) as low as 0.04–0.05 (MI is defined as the peak negative pressure divided by the square root of the ultrasonographic frequency).
When the MI is <0.1, contrast agents enter a state of linear oscillation and remain stable. When the MI >1.0 the contrast bubbles are in a state of non-linear oscillation and rupture.22 PTT and MP were measured and calculated based on previously described methods.4 Briefly, 0.03 mL/kg of an echocardiographic contrast (BR 38, provided by Bracco Research) bolus was injected for PTT analysis, while a constant-rate infusion (0.035 mL/kg/min) was used to assess MP. Using the left parasternal apical 4-chamber view, PTT was estimated based on when contrast was first visualised in the right atrium until contrast was first visualised in the left atrium. The number of beats and average heart rate between these two events were recorded in order to establish an average R-R interval and thus nPTT was calculated by dividing the PTT by this interval. Qualitative MP was measured from the left apical 4-chamber view and estimated using direct image comparison. When opacification of the myocardial contrast reached a steady state, a flash at high MI >1.0 was delivered to induce synchronous microbubble rupture, followed by an immediate return to low MI imaging during the phase of microbubble replenishment. The procedure was repeated twice for each infusion rate. At least 15 cardiac cycles after microbubble rupture were recorded and stored digitally. Images were selected as the last late systolic contraction prior to contrast capitulation and were compared side by side to images acquired after microbubble rupture in order to subjectively establish the time required for the myocardium to again be fully perfused with contrast agent (MP).
The average heart rate and number of cardiac cycles were recorded in order to establish the R-R interval and thus calculate nMP using the same method used for calculating nPTT. This study was conducted at two referral veterinary hospitals (Kansas State Veterinary Teaching Hospital, KS, USA; Dogs and Cats Veterinary Referral & ER, Bowie, MD, USA). Cardiac imaging of the normal dogs and the dogs with myxomatous mitral valve disease was performed at the same point in time. All echocardiographic images were collected by two board-certified cardiologists (MB and LBR) following previously published guidelines.23,24 All images and cine loops were digitally stored and analysed offline using dedicated software (TomTec Image Arena Browser, TomTec Imaging Systems, GmbH, Munich, DE). Echocardiographic analysis was performed by two investigators (SA and MB) blinded to treatment groups and based on previously described methods. The average value of three measurements for each variable was used for analysis.
Statistical analysis
Statistical analysis was conducted with commercially available software (JMP Pro 10, SAS Institute Inc., Cary, NC. USA). Continuous data were tested for normality by visual inspection of the data and confirmed with a Shapiro-Wilk W Test. Normally distributed data are presented as mean SD and differences between groups were assessed using an unpaired Student’s t-test for these data. Nonnormally distributed data are presented as median (min.–max.) and differences between groups were assessed using a Mann–Whitney U test. Differences among nPTT and nMP in patients at T0 and T1 were assessed using a paired Student’s t-test. To assess the reproducibility of measuring nPTT and nMP, three randomly selected datasets were analysed three times by the same observer (SA) and another six randomly selected datasets were analysed by two different observers (SA and MB). Intra- and interobserver coefficients of variation were then calculated. A level of P ≤ 0.05 was considered significant for all the tests.
Results
Healthy dogs versus dogs affected by myxomatous mitral valve disease Signalment and echocardiographic baseline data for the healthy dogs and dogs with MMVD are reported in Table 1. Healthy dogs were younger and had a higher ejection fraction and a shorter nPTT than affected dogs. There was no difference in body weight or nMP between the two groups at T0.
Dogs affected by myxomatous mitral valve disease: treated with pimobendan versus controls At baseline there were no differences in age, weight, ejection fraction and nPTT between the dogs randomised to receive pimobendan and the control dogs. In contrast, nMP was significantly lower in dogs randomised to receive pimobendan (P = 0.0315, Table 2). Comparisons of nPTT and nMP at T0 and T1 in control and pimobendan group are reported in Figure 1. Normalised PTT was lower in 5/6 dogs receiving pimobendan at T1 compared with T0 (Figure 1A), but was unchanged in 1 dog, resulting in an overall significant decrease of nPTT in dogs receiving pimobendan at T1 compared with T0 (P = 0.0250). There was no difference in nPTT at T1 compared with T0 in dogs receiving only furosemide and enalapril (P = 0.7783); in this group, data from 5 dogs only were analysed because 1 dog died before follow-up examination.
No significant difference in normalised MP was observed between T0 and T1 in the 5/6 dogs from the pimobendan group (P = 0.8798, Figure 1C) and the 4/6 dogs in the control group (P = 0.4440, Figure 1D). One dog in each group did not have images of acceptable quality for analysis and one dog in the control group died before the follow-up visit. Intra-observer coefficients of variation were 7.4% for nPTT and 7.5% for nMP, and the respective interobserver values were 13.8% and 3.8%.
Discussion
In this study we determined that nPTT, measured by contrast echocardiography, is increased in dogs with ACVIM Class C myxomatous mitral valve disease when compared with healthy dogs and that pimobendan treatment decreased nPTT in the majority of the dogs studied. Normalised MP was not significantly different between healthy dogs and dogs with MMVD and pimobendan treatment did not affect nMP in diseased dogs.
PTT has been measured using various modalities in the past and increased PTT values have been shown in dogs with MMVD, based on first-pass radionuclide angiocardiography and ECG.4,11,25 Radionuclide angiocardiography measurement has shown an increase in nPTT associated with both compensated and decompensated myxomatous mitral valve disease.11 Our study results further support that nPTT is increased in dogs with ACVIM stage C myxomatous mitral valve disease, and contrast echocardiography provides a non-invasive and affordable modality for the measurement of this variable. Increased blood volume in the lungs has been related to elevation of nPTT.11 The lungs are capable of compensating for these changes in blood flow through capillary dilation; however, these compensatory measures decrease in efficiency as pulmonary blood flow increases.26 Mitral regurgitation induced by myxomatous mitral valve disease causes a volume overload of the pulmonary veins. As the disease process worsens, more blood accumulates in the pulmonary vessels, overwhelming pulmonary compensation.27,28 Therefore, increased nPTT might be used as an indicator of MMVD severity.
As we have demonstrated, echocardiographic contrast-mediated measurement of nPTT in dogs affected by myxomatous mitral valve disease is feasible. Moreover, we found that significant changes in nPTT are associated with the disease status and that therapeutic management using
Table 1. Baseline characteristics of healthy dogs (without evidence of cardiac or systemic disease) and dogs affected by ACVIM stage C myxomatous mitral valve disease (MMVD)
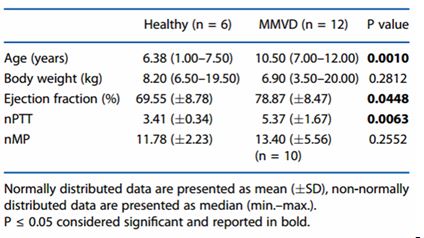
Figure 1C) and the 4/6 dogs in the control group (P = 0.4440, Figure 1D). One dog in each group did not have images of acceptable quality for analysis and one dog in the control group died before the follow-up visit. Intra-observer coefficients of variation were 7.4% for nPTT and 7.5% for nMP, and the respective interobserver values were 13.8% and 3.8%.
Discussion
In this study we determined that nPTT, measured by contrast echocardiography, is increased in dogs with ACVIM Class C myxomatous mitral valve disease when compared with healthy dogs and that pimobendan treatment decreased nPTT in the majority of the dogs studied. Normalised MP was not significantly different between healthy dogs and dogs with myxomatous mitral valve disease and pimobendan treatment did not affect nMP in diseased dogs.
PTT has been measured using various modalities in the past and increased PTT values have been shown in dogs with myxomatous mitral valve disease, based on first-pass radionuclide angiocardiography and ECG.4,11,25 Radionuclide angiocardiography measurement has shown an increase in nPTT associated with both compensated and decompensated myxomatous mitral valve disease.11 Our study results further support that nPTT is increased in dogs with ACVIM stage C MMVD, and contrast echocardiography provides a non-invasive and affordable modality for the measurement of this variable. Increased blood volume in the lungs has been related to elevation of nPTT.11 The lungs are capable of compensating for these changes in blood flow through capillary dilation; however, these compensatory measures decrease in efficiency as pulmonary blood flow increases.26 Mitral regurgitation induced by myxomatous mitral valve disease causes a volume overload of the pulmonary veins. As the disease process worsens, more blood accumulates in the pulmonary vessels, overwhelming pulmonary compensation.27,28 Therefore, increased nPTT might be used as an indicator of MMVD severity.
As we have demonstrated, echocardiographic contrast-mediated measurement of nPTT in dogs affected by myxomatous mitral valve disease is feasible. Moreover, we found that significant changes in nPTT are associated with the disease status and that therapeutic management using pimobendan was reflected in a significant reduction of nPTT in the majority of the dogs in this study. Thus, echocardiographic contrastmediated measurement of nPTT might have a future role in the clinical management of dogs with myxomatous mitral valve disease.
Table 2. Baseline (T0) characteristics of dogs affected by myxomatous mitral valve disease (MMVD) ACVIM stage C, all receiving enalapril and furosemide, and randomised to receive pimobendan or not (Control dogs)
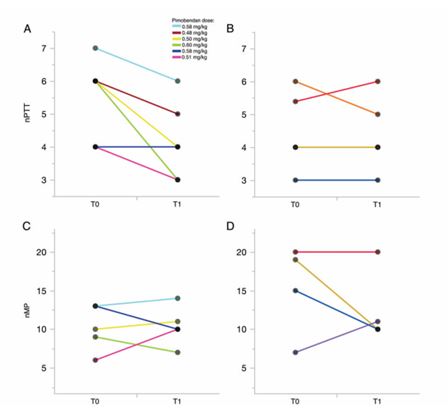
Figure 1. Pulmonary transit times (nPTT) and myocardial perfusion (nMP), normalised for heart rate, at T0 (baseline) and T1 (after 1 week) in a population of dogs (n = 12) with myxomatous mitral valve disease
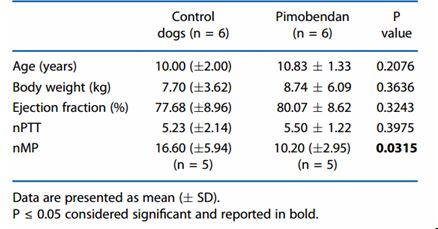
Dogs were treated with pimobendan (n = 6) or given no treatment (n = 6). The difference in values at T0 vs T1 was assessed using a paired Student’s t-test. Change in nPTT values in (A) dogs receiving pimobendan (P = 0.025) and (B) dogs not receiving pimobendan (P = 0.778). Change in nMP values in (C) dogs receiving pimobendan (P = 0.880) and (D) dogs not receiving pimobendan (P = 0.444). Lines of the same colour represent the same dog and the pimobendan dose for that dog.
It is hypothesised that dogs with MMVD have some degree of myocardial fibrosis and thus an increase in MP times.10 This parameter may therefore be used to estimate the amount of myocardial fibrosis and may, if further characterised, provide additional information about MMVD severity. In our study, there was no difference in MP in dogs affected with myxomatous mitral valve disease compared with healthy dogs. Although in previous studies pimobendan has been shown to improve MP in dogs, using radionuclide-labelled microspheres and a gamma counter,15 in the current study MP did not decrease when pimobendan was added to the treatment. The inability to detect any difference in nMP in this study could be because the disease and ensuing fibrosis were not significant enough to reduce nMP in this population of dogs, resulting in an inability to detect an improvement in nMP following pimobendan therapy. If this is the case, we are unable to make any conclusion about the effects of pimobendan on nMP in this dog population.
Another possible explanation is that the changes in MP induced by myxomatous mitral valve disease are generally not significant enough to be detected using contrast-mediated nMP measurement in dogs. It is also possible that contrast-mediated measurement of MP is not sensitive enough to detect changes in MP associated with pimobendan therapy. Theoretically, pimobendan should improve cardiac contractility and decrease coronary artery and myocardial capillary vascular resistance, thereby improving nMP. As this response has been shown in healthy hearts, it is reasonable to postulate that diseased hearts may behave similarly.11
It is also possible that the diseased heart responds differently to pimobendan and thus nMP may not improve in dogs with myxomatous mitral valve disease. The follow-up assessment in our study was at 1 week. Although this is likely to be enough time to demonstrate a haemodynamic improvement such as in nPTT, it can be too short for identifying any significant changes in myocardial fibrosis. Additionally, although not yet reported for pimobendan, individual variability in the response to this drug could be related to individual pharmacogenetic differences. It is also possible that our small study did not have enough statistical power to show significant alterations of nMP in the dogs with myxomatous mitral valve disease. The observed variation of nMP in some individual dogs can also be related to inter-day variability of this measurement. Despite being a non-invasive procedure, echocardiography can induce stress in dogs, with associated coronary vasoconstriction, and individual dogs may present different responses to stress and this can be responsible for changes or lack of changes observed. Moreover, despite our attempts to create a homogeneous population, individual diseased dogs in the pimobendan group had a significantly lower nMP at T0. Although these dogs were all classified as stage C MMVD, this finding suggests that fibrosis could be worse in the no-pimobendan group. Moreover, despite being in the same class of heart failure, the degree of myocardial fibrosis can differ among individuals.9 A larger study is required to make any conclusions regarding nMP in dogs with myxomatous mitral valve disease, while a randomised control study design for dogs with increased nMP could provide additional information about pimobendan treatment.
In conclusion, measurement of nPTT and nMP by contrast echocardiography was feasible in dogs affected by ACVIM stage C MMVD.
In our study, dogs with stable ACVIM stage C myxomatous mitral valve disease had an increased nPTT compared with healthy dogs. Pimobendan treatment decreased nPTT in most of the dogs with MMVD, in agreement with previous studies.29 Although MP was unchanged in the group of dogs with myxomatous mitral valve disease, this parameter varied significantly, suggesting that this group of dogs was heterogeneous and myocardial fibrosis was clinically relevant in some patients. Results from this pilot study should encourage the performance of a larger study.
References
- Bilotta F, Agati L, Fiorani L et al. Pulmonary transit of echocontrast agents during mechanical ventilation: a clinical transthoracic echocardiographic study. Echocardiography 2005;22:395–401.
- Dijkmans PA, Juffermans LJ, Musters RJ et al. Microbubbles and ultrasound: from diagnosis to therapy. Eur J Echocardiogr 2004;5:245–256.
- Rakhit DJ, Becher H, Monaghan M et al. The clinical applications of myocardial contrast echocardiography. Eur J Echocardiogr 2007;8:S24–29.
- Crosara S, Ljungvall I, Margiocco ML et al. Use of contrast echocardiography for quantitative and qualitative evaluation of myocardial perfusion and pulmonary transit time in healthy dogs. Am J Vet Res 2012;73:194–201.
- Streitberger A, Hocke V, Modler P. Measurement of pulmonary transit time in healthy cats by use of ultrasound contrast media “Sonovue(R)”: feasibility, reproducibility, and values in 42 cats. J Vet Cardiol 2013;15:181–187.
- Porter TR, Xie F. Myocardial perfusion imaging with contrast ultrasound. JACC Cardiovasc Imaging 2010;3:176–187.
- Santalo M, Martin A, Velilla J et al. Using high-sensitivity troponin T: the importance of the proper gold standard. Am J Med 2013;126:709–717.
- O’Brien PJ, Smith DE, Knechtel TJ et al. Cardiac troponin I is a sensitive, specific biomarker of cardiac injury in laboratory animals. Lab Anim 2006;40:153–171.
- Falk T, Ljungvall I, Zois NE et al. Cardiac troponin-I concentration, myocardial arteriosclerosis, and fibrosis in dogs with congestive heart failure because of myxomatous mitral valve disease. J Vet Intern Med 2013;27:500–506.
- Falk T, Jonsson L, Olsen LH et al. Arteriosclerotic changes in the myocardium, lung, and kidney in dogs with chronic congestive heart failure and myxomatous mitral valve disease. Cardiovasc Pathol 2006;15:185–193.
- Lord P, Eriksson A, Haggstrom J et al. Increased pulmonary transit times in asymptomatic dogs with mitral regurgitation. J Vet Intern Med 2003;17:824–829.
- Boyle KL, Leech E. A review of the pharmacology and clinical uses of pimobendan. J Vet Emerg Crit Care 2012;22:398–408.
- Fuentes VL. Use of pimobendan in the management of heart failure. Vet Clin North Am Small Anim Pract 2004;34:1145–1155.
- Takeda N, Hayashi Y, Arino T et al. Effect of pimobendan in patients with chronic heart failure. Exp Clin Cardiol 2001;6:195–199.
- Pagel PS, Hettrick DA, Warltier DC. Influence of levosimendan, pimobendan, and milrinone on the regional distribution of cardiac output in anaesthetized dogs. Br J Pharmacol 1996;119:609–615.
- Haggstrom J, Boswood A, O’Grady M et al. Effect of pimobendan or benazepril hydrochloride on survival times in dogs with congestive heart failure caused by naturally occurring myxomatous mitral valve disease: the QUEST study. J Vet Intern Med 2008;22:1124–1135.
- Suzuki S, Fukushima R, Ishikawa T et al. The effect of pimobendan on left atrial pressure in dogs with mitral valve regurgitation. J Vet Intern Med 2011;25:1328–1333.
- Atkins C, Bonagura J, Ettinger S et al. Guidelines for the diagnosis and treatment of canine chronic valvular heart disease. J Vet Intern Med 2009;23:1142–1150.
- Cornell CC, Kittleson MD, Della Torre P et al. Allometric scaling of M-mode cardiac measurements in normal adult dogs. J Vet Intern Med 2004;18:311–321.
- Hansson K, Haggstrom J, Kvart C et al. Left atrial to aortic root indices using two-dimensional and M-mode echocardiography in cavalier King Charles spaniels with and without left atrial enlargement. Vet Radiol Ultrasound 2002;43:568–575.
- Schiller NB, Shah PM, Crawford M et al. Recommendations for quantitation of the left ventricle by two-dimensional echocardiography: American Society of Echocardiography Committee on Standards, Subcommittee on Quantitation of Two-Dimensional Echocardiograms. J Am Soc Echocardiogr 1989;2:358–367.
- Serra V, Fernandez MA, Zamorano JL. Microbubbles: basic principles. In: Zamorano JL, Fernandez MA, editors. Contrast echocardiography in clinical practice. Springer-Verlag Mailand, Milan, 2004:19–43.
- Thomas WP. Two-dimensional, real time echocardiography in the dog: technique and anatomic validation. Vet Radiol 1984;25:50–64.
- Bonagura JD. M-mode echocardiography: basic principles. Vet Clin North Am Small Anim Pract 1983;13:299–319.
- Muller HM, Rehak PH, Puchinger M et al. Measurement of cardiac output and pulmonary transit time for assessment of pulmonary vascular resistance in domestic piglets. Exp Physiol 2009;94:659–664.
- Presson RG Jr, Hanger CC, Godbey PS et al. Effect of increasing flow on distribution of pulmonary capillary transit times. J Appl Physiol 1994;76:1701–1711.
- Fox PR. Pathology of myxomatous mitral valve disease in the dog. J Vet Cardiol 2012;14:103–126.
- Kellihan HB, Stepien RL. Pulmonary hypertension in canine degenerative mitral valve disease. J Vet Cardiol 2012;14:149–164.
- Haggstrom J, Lord PF, Hoglund K et al. Short-term hemodynamic and neuroendocrine effects of pimobendan and benazapril in dogs with myxomatous mitral valve disease and congestive heart failure. J Vet Intern Med 2013;27:1452–1462.
^Наверх